project experiment
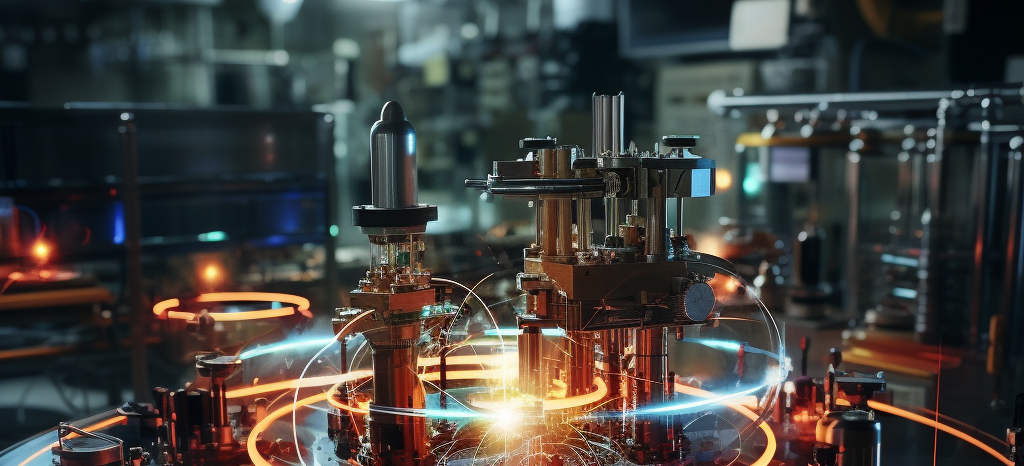
Principal Investigator: Michael Epperson. Co-Investigators: Elias Zafiris, Senior Research Fellow in Theoretical and Mathematical Physics, University of Athens; Stuart Kauffman, Research Professor, Complex Systems Center, University of Vermont; Timothy Eastman, NASA-Goddard; Phillip Stamp, Professor of Physics, University of British Columbia; Karim Bschir, Swiss Federal Institute of Technology. Consultants: David Finkelstein, Professor Emeritus of Physics, Georgia Institute of Technology; Roland Omnès, Laboratoire de Physique Théorique Université de Paris XI, (Unité Mixte de Recherche, CNRS). Supported by a grant from the Fetzer-Franklin Fund (Grant D21C62)
PUBLICATION DELIVERABLE
Stamp, P., Takahashi, S. et al. “Decoherence in crystals of quantum molecular magnets.” Nature 476.7358 (2011): 76-79
BACKGROUND
Interpretations of quantum physics such as those offered by Omnès and Griffiths derive the logical order of classical causality, in part, from the decoherence effect, whereby potential facts constitutive of a quantum mechanical system are logically ordered into potential, mutually consistent histories. Decoherence is thus given by these interpretations as a derivation of classical logical causality from the quantum mechanical correlation of the causal and logical orders. And indeed, there have been experiments by which the logical order of classical causality can be seen as deriving from the logical integrations of potentia yielded by decoherence. Caldeira and Leggett (1983) created a successful demonstration of such a derivation. Using the classical Lorentz oscillator model, they showed that the quantum interferences manifest by the oscillations were cancelled out via the decoherence effect. The latter, in other words, can be seen as introducing logical constraints upon the quantum system. Given sufficient time for decoherence to occur, the system becomes describable as a classical probability distribution in phase space. Moreover, observable consequences of co-investigator David Finkelstein’s theory (as characterized, for instance, in Finkelstein et al., [2001] “Clifford Algebra as Quantum Language,” J. Math. Phys 42, 1489-1502) are discussed in the model of the oscillator in Finkelstein and Shiri-Garakani, 2004c Finite Quantum Harmonic Oscillator.
Even more significant, recent experiments on precessional non-dissipative decoherence in spin bath dynamics are arguably even better at exemplifying the concept of ‘logical conditioning of potentia’ in the relational realist interpretation of quantum mechanics; for unlike the oscillator bath and other environmental decoherence models, the phenomenon of precessional decoherence entails no energy transfer between the bath and the measured system. That is to say, non-dissipative precessional, intrinsic, and ‘third party’ decoherence which we propose to investigate in this continuation of our project, cannot be simply reduced to the dynamics of efficient physical causality—the ‘causally closed’ framework environmental decoherence has typically been fitted to.
The fundamental question we will be exploring, then, is whether there are intrinsic sources of decoherence in Nature—i.e., sources that cannot be accounted for, in an experimental setting, solely via any of the known decoherence mechanisms. Experimental investigation of the hypothesis of intrinsic decoherence is as crucial to the enterprise of philosophy as it is to the enterprise of physics; for the implication is that decoherence, and the associated logical conditioning of physical causality by which the effects of decoherence are recognized, is inevitable. It does not, in other words, merely arise as an epiphenomenon from dissipative, purely efficient causal processes, as has been the popular understanding of environmental decoherence for a number of years. Dissipation and decoherence are indeed tied together in the oscillator bath models of the environment; but as mentioned above, and discussed further below, this result is not true for spin baths, where one can have decoherence with no dissipation, even at T = 0; and in the case of third-party decoherence there cannot possibly be any environmental dissipation, at any T, since there is no direct coupling to the environment. Thus there is no necessary connection between decoherence and dissipation in the real world, and no necessary reason for it to cease at T = 0. This is a problem of real practical interest right now, both for the construction of quantum information processing systems and for the standard physics of solids, and there is consequently a substantial worldwide quest underway exploring ways to eliminate environmental decoherence. Thus the question of ‘intrinsic’ sources of decoherence in Nature, impossible to eradicate, is crucial.
The hallmark feature of the concept of intrinsic decoherence is that it is inevitable—perhaps even arising as part of the basic structure of the universe. And thus the logical conditioning of physical causality, as evinced by the effect of decoherence upon quantum systems, can be envisioned similarly—as part of the basic structure of the universe (and perhaps part of the answer to the question of why the universe is accessible to reason in the first place.) Such intrinsic sources of decoherence in Nature, operating even at T = 0, would not only provide a way of explaining the ‘emergence of classical physics’ in fields ranging from quantum cosmology to condensed matter physics; they would also place a fundamental limit on the observability of quantum phenomena. This would limit the possibility of seeing macroscopic quantum phenomena, and also place fundamental limits on the superpositions required for quantum computing.
Possibilities for intrinsic decoherence mechanisms have already emerged from both low and high-energy physics, and this is currently one of the most exciting frontiers of physics. Crucial experiments are required such as the one proposed herein. But if fitness is to be tested and evaluated among competing physical-metaphysical interpretations of quantum mechanics—i.e., those that dualistically treat actuality & the causal order and potentiality & the logical order as separate or separable features of reality, versus the relational realist interpretation, which treats actuality and potentiality as dipolar, mutually implicative features of every quantum praxis event—it is equally crucial that the conception of experiment be sufficiently free of serious constraints imposed by any particular ontological commitment. This is especially important with respect to certain of these commitments that enjoy the status of ‘convention.’ Thus an emphasis on experimental testing, such as those discussed above, combined with reduced model-dependence, and a turn away from the typical conditioning influence of traditionally inherited ontological presuppositions, will be an important prescription for the development of metaphysically coherent interpretations of physical theories such as quantum physics. The EPR gedanken experiment, for example, was conceived by its authors via the conventional, inherited classical mechanistic-materialistic ontology. But more recent EPR-like tests of quantum nonlocality, rather than being conceived as constricted to this ontology, were conceived to test the limitations of this ontology.
EXPERIMENT
The experimental component of this project culminated in the publication of Stamp, P., Takahashi, S. et al. “Decoherence in crystals of quantum molecular magnets.” Nature 476.7358 (2011): 76-79. This paper by project Co-I Philip Stamp, was a follow-up to recent work by S. Bertaina, B. Barbara, et al. (“Quantum Oscillations in a Molecular Magnet,” Nature, 453, 8 May 2008. See also, Stamp’s discussion of this experiment on page 167 of the same issue.)
This work built upon recent groundbreaking experiments on the mechanisms of decoherence in molecular magnets. In Bertaina et al., three decoherence mechanisms were accounted for: Spin bath, lattice vibrations, and dipolar pairwise decoherence. The observed coherence time was 800 ns for the spin ½ transitions of the Vanadium molecule. It is proposed that if the experiment were performed at liquid He temperatures, with spin-free molecules, sufficiently separated by a surfactant to restrict dipolar pairwise decoherence, coherence time could be extended to 100 microseconds. Our own experimental work tests this and related hypotheses.
This experimental investigation is crucial for the relational realist interpretation of quantum mechanics, and its conception of logical causality, because spin-bath / precessional decoherence involves no transfer of energy. It is entirely non-dissipative, unlike the more common oscillator bath models that have been used in previous experiments. Non-dissipative decoherence is arguably something 'deeper' than dissipative environmental decoherence via simple efficient physical causality, which is the framework environmental decoherence has typically been fitted to. Our goal, then, is to develop an experiment that attempts to differentiate between precessional / spin bath decoherence and other possible sources of 'intrinsic' decoherence, so that the latter can be further elucidated.
Thisl work will help shed light on, 1. how decoherence can be examined as a potential challenge to (or refinement of) Leggett's thesis of macrorealism (which would be a much needed blow to the conventional mechanistic-materialistic paradigm); and 2. how decoherence—especially non-dissipative decoherence seen in the spin bath, 3rd party, and 'intrinsic' conceptions—can be interpreted as a physical exemplification of 'logical causality' via ‘logical-relational decoherence.’ In this regard, decoherence might be seen as implying a logically governed conditioning of physical causality at the level of microphysical reality—one that could account for the tacitly presupposed correlation of the causal and logical orders at the classical level as an emergent phenomenon.
Since the correlation of the causal and logical orders has been a centuries-old problem in natural philosophy, the obvious relevance of the logical order to decoherence warrants careful study. Possible implications include new understandings of: 1. the quantum measurement problem, 2. the supposed quantum-classical boundary, 3. relating the asymmetrical logical order to the asymmetrical cosmological arrow, thermodynamic arrow, and quantum arrow (to the extent that the latter is asymmetrical, for example, via Von Neumann’s Process 1); and 4, the conception of classical physics as ‘emergent’ from quantum physics, via the decoherence effect.
With respect to the latter, one might focus on the more physical question of how classical quasi-deterministic behavior emerges for large systems, and/or how quasiclassical stochastic behavior emerges, even for small systems. Co-investigator Philip Stamp writes, “a proper answer to this question requires understanding the real decoherence mechanisms operating in Nature and that these are not so simple, or necessarily completely understood. Thus we do not yet have a theory which derives classical physics from quantum physics solely using ideas from decoherence, even though we do have some derivations of classical behaviour within certain models.”
The best systems to initially do decoherence tests in are thus the ones (i) in which one has a large number of degrees of freedom, and (ii) in which decoherence processes are understood best. The only systems that have so far shown agreement between experiment and theoretical predictions, and which involve large numbers of degrees of freedom, are large-spin magnetic molecules. Those that have been tested are the V-15 molecule (Bertaina et al., Nature 2008), and the Fe-8 molecules (Takahashi et al., preprint about to be issued); in both cases, experiment agrees with theoretical prediction to within a relatively small experimental error. The Fe-8 molecule has over 10^{50} degrees of freedom in the nuclear bath; the low-T quantum dynamics of this system is entirely governed by the coupled dynamics of the electron spins with these nuclear spins, with the phonon bath, and with other molecules (with photons playing a secondary role).
In unpublished work the Bertaina-Barbara group has now also checked out the role of dipolar interactions between the V-15 molecules, by varying the distance separating the molecules. Theory and experiment still agree. In both of these systems (and also in related systems like the Erbium tungstenates, or the LiHoF system, where one deals with a large Rare Earth spin, and one also has a very good handle on the experimental systems [here the number of degrees of freedom is much smaller however]), one can therefore look for a key signature of ‘intrinsic decoherence,’ where it is most likely to show up: in the dynamics of decoherence once entanglement has been set up between 2 or more of these molecules.